In Vivo Fluorescence Imaging in the NIR-II Spectral Region for Early Cancer Detection
Introduction
UV, VIS, and NIR-I detection methods have been used in various scientific and medical applications for decades. Each of these approaches, however, has its limitations. For instance, light at UV and visible wavelengths is quite easily detectable using silicon-based CCD technologies but is unable to penetrate samples due to reflection and scattering.
New CCD cameras can detect NIR-I wavelengths from 750 nm up to almost 1100 nm and thus provide slight penetration into samples. Unfortunately, above this threshold the silicon is transparent to light. Such CCD cameras have found limited use in drug discovery applications.
The NIR-II window, whose wavelength range extends to 1700 nm, achieves much deeper sample penetration (see Figure 1). Spurred on by the development of InGaAs and InSb detectors, the NIR-II, also known as the shortwave-infrared or SWIR, has opened up a new world of scientific and medical applications.
Figure 1: NIR-I vs. NIR-II: resolution and penetration depth. Images and graph courtesy of Dr. Dominik J.Naczynski, Stanford University, adapted from Lim, Y.T. et al. Mol Imaging. (2003) 2, 50.
As many research groups around the world are developing and evaluating new NIR-II probes
appropriate for small-animal imaging in preclinical research, scientists now begin to focus more
on the clinical applications for disease detection, morphology, and drug detection in human patients
NIR-II Fluorescence Endoscopy for Imaging of Colorectal Cancer
Recently, a multidisciplinary collaborative research team in China and the United States designed, constructed, and characterized an endoscopic system to perform targeted NIR-II fluorescence imaging of colorectal cancer [1]. The team used the new NIR-II endoscopy imaging system to evaluate a synthesized fluorescent molecular probe, indocyanine green (ICG) conjugated bevacizumab (Bev-ICG), which targets vascular endothelial growth factor (VEGF), an important biomarker that is overexpressed in most colorectal cancers.
The study was spearheaded by the research group of Dr. Hongguang Liu at the Institute of Molecular Medicine Joint Laboratory for Molecular Medicine at Northeastern University in Shenyang, Liaoning, and supported in part by the Natural Science Foundation of China, the Clinical Capability Construction Project for Liaoning Provincial Hospitals, and a China postdoctoral science foundation grant.
The researchers note that while endoscopy is a clinical gold standard for examining the interior of a hollow organ or body cavity, they are the first to report the use of NIR-II fluorescence endoscopy for the targeted detection of colorectal cancer. Their innovative endoscopic imaging system, which enables the simultaneous acquisition and display of white light (WL) and NIR-II fluorescence, delivers subcellular resolution of 20 μm for sharp images in the NIR-II region. A scientific InGaAs camera from Teledyne Princeton Instruments was used for the realtime, noninvasive, in vivo NIR-II imaging of colorectal tumor biomarkers (see Figure 2).
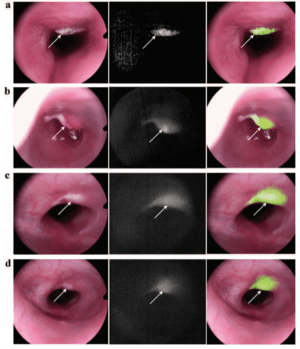
Figure 2: Simultaneous WL and fluorescence images of representative tumors.
(a, b) The tumors that were visible in WL show clearly increased fluorescence.
(c, d) Representative images of the tumors that are easy to miss in WL images
but are obvious in fluorescence imaging. Images courtesy of Dr. Hongguang Liu
(Institute of Molecular Medicine Joint Laboratory for Molecular Medicine,
Northeastern University, Shenyang, Liaoning, China). First published in Adv.
Healthcare Mater. 2019, 1900974.
Unlike NIR-I fluorescence imaging, which uses visible light for excitation, the excitation wavelength for NIR-II fluorescence imaging is typically in the (invisible) NIR-I range. Therefore, the conventional endoscopy imaging process does not need to be interrupted to collect the NIR-II fluorescent data. The NIR-II fluorescence image can be generated and displayed simultaneously with the WL image to afford researchers real-time visualization of tissue morphology and molecular characteristics from fluorescent probes. The future utilization of molecular probes with stronger fluorescence emission in the NIR-II region is expected to further improve the sensitivity of the NIR-II endoscopy system for tissue characterization.
By design, the new system can be easily adapted to endoscopy systems that are equipped with a standard coupling. The researchers anticipate similar hardware upgrades will greatly promote the application of NIR-II fluorescence imaging in clinical settings.
NIR-II Fluorescence Imaging of Circulatory and Skeletal Systems
Dr. Zhen Cheng, an associate professor of radiology at Stanford University, is a member of the collaborative research team responsible for the NIR-II endoscopy system discussed above. Dr. Cheng's laboratory at Stanford seeks to develop novel molecular imaging probes and techniques for noninvasive detection of cancer and its metastasis at the earliest stage.
The techniques developed via Dr. Cheng's research enable a close examination of the molecular, metabolic, and physiological characteristics of cancers and their responses to therapy. His lab identifies novel cancer biomarkers with significant clinical relevance, develops new chemistry for probes preparation, and validates new strategies for probes high-throughput screening using NIR-II fluorescence imaging [2-6].
In a recent project, Dr. Cheng and his colleagues performed in vivo imaging of the circulatory system comparing various NIR-II organic small-molecule probes, CQ-T (CQ-1-4T), that were designed in the lab and loaded with biocompatible human serum albumin (HSA) to improve quantum yield [2]. The experiments recorded emission spectra, quantum yield, and in vivo NIR-II images using an InGaAs camera from Teledyne Princeton Instruments (see Figure 3).
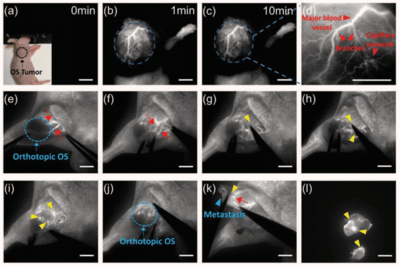
Figure 3: In vivo NIR-II imaging for vascular network of tumor and surgical navigation. (a-c) In vivo NIR-II fluorescence
imaging performed on nude mice with xenograft osteosarcoma on day 14 and recorded within 10 min after CQL injection
intravenously, respectively. (d) High magnification of (c) and red arrowheads showing the abundant irregular branches and
capillary network of tumor-supporting blood vessels. (e, f) Nude mice with femur orthotopic osteosarcoma was employed
and the skin around the tumor was incised shortly after CQL was injected. The tumor blood supply branches (red arrows)
were exposed from femoral artery. (g-j) A vessel clamp was implemented to induce the temporary block of major tumor-
supporting blood vessels with ligation and dissection of the tumor-supporting blood branches. Yellow arrowheads
indicated the embolization induced by vessel clamp. (k) The blood supply of tumor metastasis stemming from abdominal
artery (red arrow) and the embolization induced by vessel clamp (yellow arrowhead). (l) Ex vivo NIR-II image of ligated
and dissected orthotopic osteosarcoma and metastasis. Scale bar: 4.0 mm. Images courtesy of Dr. Zhen Cheng,
Stanford University. First published in Adv. Funct. Mater. 2019, 1906343.
Among the series of probes evaluated, the researchers found one, CQL (CQ-4T/HSA), that demonstrates superior optical properties and a 6.65x increase in fluorescence compared to the small molecule alone. Utilized in concert with the noninvasive, nonradiation NIR-II imaging modality, CQL enables multifunctional imaging for visualizing and monitoring circulatory system-related physiological and pathological processes in vivo, including thrombosis, peripheral arterial disease, tumor angiogenesis, and lymphatic drainage.
During NIR-II imagenavigated surgery, CQL is capable of helping physicians implement precise intervention of tumor and sentinel lymph node biopsy more accurately with less side effects. The researchers conclude that high resolution, excellent biocompatibility, and favorable in vivo performance make CQL a promising candidate for further preclinical applications and future translations into clinical practices.
Another recent study from Dr. Cheng's lab investigated a NIR-II fluorophore based on DSPEmPEG encapsulated rare-earth-doped nanoparticles, RENPs@DSPE-mPEG, that shows inherent affinity to bone without linking any targeting ligands (see Figure 4) [3].
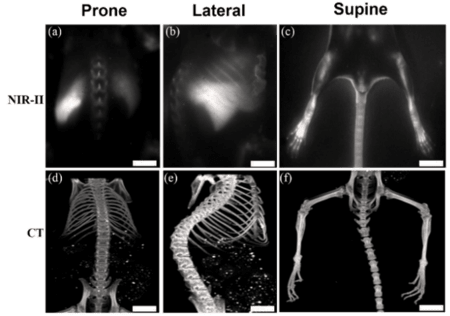
Figure 4: NIR-II imaging of C57BL/6 mice (n=3) bone in the NIR-IIa 1345 nm (1250 nm long-pass filter,
exposure time 1000 ms) windows (a, b, and c) and corresponding CT imaging (d, e, and f).
Scale bar: 10 mm. Images courtesy of Dr. Zhen Cheng, Stanford University. First published in Nano. Lett.
2019 May 8;19(5):2985-2992.
This noninvasive, nonradiation strategy for skeletal system mapping and bone disease diagnoses is also applicable to blood vessels and lymph nodes. Significantly, RENPs@DSPEmPEG can be internalized by circulating white blood cells, which may increase efficient nanoparticle delivery for immunotherapy as well as improve the diagnostic and therapeutic efficacy of cancer-targeted nanoparticles in clinical applications.
Enabling Technology
The NIRvana® family of InGaAs cameras from Teledyne Princeton Instruments differentiates itself from other InGaAs cameras via a number of scientific performance features, including deep cooling, low dark noise, high linearity, low read noise, high frame rates, intelligent software, and precision control over integration times.
First and foremost, maintenance-free thermoelectric cooling (not liquid nitrogen) chills the NIRvana 640 camera's InGaAs detector down as low as -85°C. This deep cooling is coupled with a proprietary cold shield design and vacuum technology to facilitate the lowest possible dark noise, which helps increase sensitivity as well as preserve signal-to-noise ratio (SNR) for
long exposure times.
The NIRvana 640 camera has the ability to expose for as short as 2 μs, up to many minutes. Ultra-low-noise readout electronics help ensure good SNR even when the camera is operated at its maximum rate of 110 full frames per second. Furthermore, excellent camera linearity means that it is highly reliable for medical research.
Teledyne Princeton Instruments' 64-bit LightField® software, available as an option, provides a powerful yet easy-to-use interface that puts real-time online processing capabilities at the researcher's fingertips. NIRvana cameras can also be integrated into larger experiments using an available National Instruments LabVIEW® toolkit. Full triggering support is provided for synchronization with external equipment.
Acknowledgments
Teledyne Princeton Instruments would like to thank Dr. Hongguang Liu and Dr. Zhen Cheng for their invaluable contributions to this application note.
Resources
To learn more about the work of Dr. Zhen Cheng's laboratory at Stanford University, please visit: https://profiles.stanford.edu/zhen-cheng?tab=bio
References
- Suo Y., Wu F., Xu P., Shi H., Wang T., Liu H., Cheng Z. NIR-II fluorescence endoscopy for targeted imaging of colorectal cancer. Adv. Healthcare Mater. 2019, 1900974.
- Li D., Qu C., Liu Q., Wu Y., Hu X., Qian K., Chang B., He S., Yuan Y., Li Y., Ko T., Yu A., Cheng Z. Monitoring the real-time circulatory system-related physiological and pathological processes in vivo using a multifunctional NIR-II probe. Adv. Funct. Mater. 2019, 1906343.
- He S., Chen S., Li D., Wu Y., Zhang X., Liu J., Song J., Liu L., Qu J., Cheng Z. High affinity to skeleton rare earth doped nanoparticles for near-infrared II imaging. Nano. Lett. 2019 May 8;19(5):2985-2992.
- Ning Y., Chen S., Chen H., Wang J., He S., Liu Y., Cheng Z., Zhang J. A proof-of-concept application of water-soluble ytterbium(iii) molecular probes in in vivo NIR-II whole body bioimaging. Inorg. Chem. Front. 2019; 6(8):1962-1967.
- Li D., He S., Wu Y., Liu J., Liu Q., Chang B., Zhang Q., Xiang Z., Yuan Y., Jian C., Yu A., Cheng Z. Excretable lanthanide nanoparticle for biomedical imaging and surgical navigation in the second near-infrared window. Adv. Sci. (Weinh.) 2019; 6(23):1902042.
- Qian K., Qu C., Ma X., Chen H., Kandawa-Schulz M., Song W., Miao W., Wang Y., Cheng Z. Tuning the near infrared II emitting wavelength of small molecule dyes by single atom alteration. Chem. Commun. (Cambridge, U. K.) 2019.