Fluorescence Recovery After Photobleaching (FRAP)
Introduction
Fluorescent markers become excited by specific wavelengths of light, and then emit light in a different wavelength. This makes them very valuable for scientific research in order to locate cells/proteins and even track them over time. However, if a very intense light is used to excite the fluorophores, or they are exposed for too long, the fluorescent molecules lose their ability to emit light and are referred to as bleached or photobleached. Bleached fluorophores permanently lose their ability to fluoresce, the process is an irreversible photochemical alteration. This is a big issue in imaging, if fluorophores become bleached they can no longer act as markers and imaging cannot take place. However, certain techniques take advantage of bleaching, the main one being ‘fluorescence recovery after photobleaching’, or FRAP.
Principle of FRAP
FRAP is a technique developed by Axelrod et al. (1976) as a method to study protein mobility in living cells. In FRAP, a specific area of a cell or tissue is photobleached by intense laser light, removing fluorescence from this area. This area is typically a cell membrane or area where diffusion occurs, such as the nucleus, as FRAP requires fluorescent molecules to move around freely in order to function. Fluorescence in the bleached area will slowly recover as bleached fluorophores move out and healthy fluorophores from other areas move in, hence the name fluorescence recovery after photobleaching. This process can be seen in Fig.1.
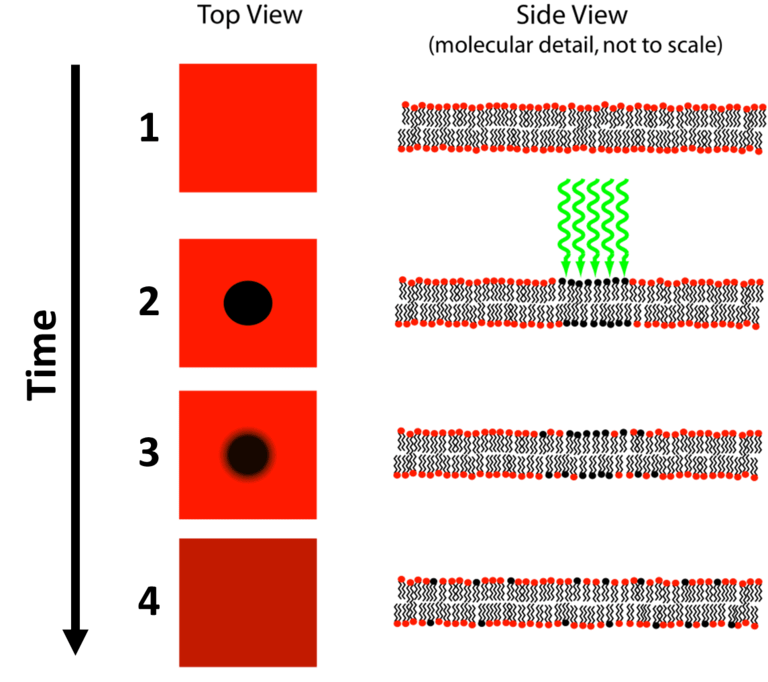
Figure 1: The principle of FRAP on a cell membrane bilayer, with a generalized top view and a molecular side view. 1) Membrane
is labelled with fluorescent molecule (red). 2) A focused laser (green) bleaches a select area of the membrane (black).
3) Diffusion of fluorescent molecules results in fluorescence recovery over time. D) After a time, fluorescence fully recovers,
but at a lower overall intensity than before. Image from Wikimedia Commons, Frap diagram.
The speed of fluorescence recovery of the bleached area is directly proportional to the diffusion speed of fluorescent or labeled molecules, which gives information about the mobility of the labelled molecules (Ishikawa-Ankerhold et al. 2012). By bleaching a component or organelle within a cell (such as the nucleus), you can then observe the rate of diffusion of molecules in and out of that component as the fluorescence recovers.
FRAP Applications
FRAP was originally developed as a method to study protein mobility in living cells and has since grown to become a popular technique for investigating molecular transport events such as diffusion, protein dynamics, or interactions between cellular components. FRAP continues to be used for protein mobility and molecular diffusion studies but has also evolved to include applications such as binding dynamics (Groeneweg et al. 2014) as well as being used in combination with single-molecule tracking (Tran-Ba et al. 2015). An example of FRAP can be seen in Fig.2.
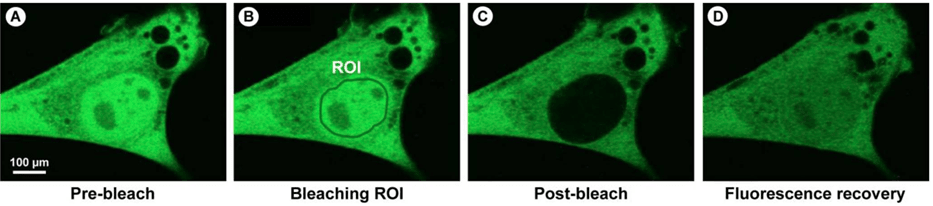
Figure 2: An example of FRAP in action. Images show a myo3 cell expressing GFP-myosin III. A) Cell is homogenously stained before bleaching occurs. B) The region of interest (ROI), in this case the nucleus, is selected. C) A high-powered laser bleaches the nucleus and the fluorophores in this area no longer emit light. D) Fluorescence in the ROI recovers through diffusion of non-bleached fluorophores, there is a decrease in the total amount of fluorescence when compared to A. Image from Ishikawa-Ankerhold et al. (2012).
FRAP was originally developed with the intention to study lipid molecules in a cell membrane, such as their motility and organization. Naturally, FRAP enjoys widespread use in the study of both biological and artificial membranes, identifying ligand binding sites and understanding cellular transduction.
However, other uses for FRAP have been identified such as for studying protein binding, including protein-protein interactions and protein trafficking. These studies show which ligands can bind to which proteins, as well as the speed of binding. By fusing fluorescent proteins such as GFP to proteins of interest, their binding mechanics can be investigated, and the ability to diffuse from one cellular compartment to another. This also crosses over with membrane studies, as protein binding interactions with the membrane can also be observed using FRAP.
FRAP also has applications beyond the membrane, as a powerful tool when studying diffusion and binding within specific areas of the cell. Numerous areas can be bleached per FRAP experiment, allowing for more complex data to be gathered about particular regions of different cell types.
Inverse FRAP (iFRAP)
Some experiments use an inverted version of FRAP, called iFRAP. This technique involves bleaching the entire cell, except the region of interest. As a much larger area of the cell is bleached, iFRAP is quite a time-consuming technique but is very useful when studying diffusion kinetics of molecules within an immobile intracellular structure. iFRAP was developed by Dundr et al. (2002) and is particularly useful when studying molecules in small organelles and how they are exchanged with the surrounding cytoplasm. An example of iFRAP can be seen in Fig.3.
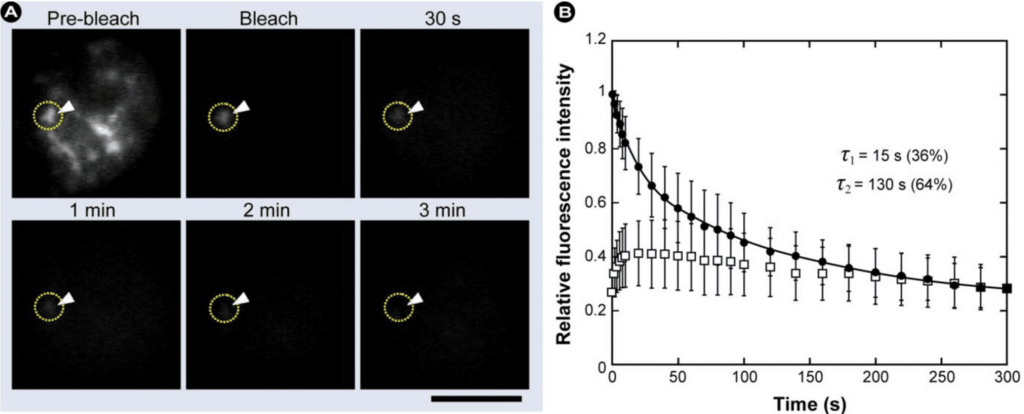
Figure 3: An iFRAP experiment on a cell. A) A Cos7 cell before bleaching, and then entirely bleached except the ROI shown in yellow. This fluorescence rapidly decreases as the fluorophores diffuse out into the cytoplasm. Scale: 10 μm. B) Quantified change in fluorescence intensity for the ROI (black square) and an adjacent area (white square) over time. Image from Ishihama et al. (2008).
Issues with FRAP
While a flexible technique for research, there are some potential issues to consider when using FRAP. Firstly, FRAP is most often used on living cells that have the capability of molecular diffusion, but these living cells also move themselves, making it challenging to pin-point and track a certain ROI that requires bleaching. In addition, these samples are 3D in nature, and fluorophores above and below the ROI focal plane are also bleached by the laser, meaning the bleached area is more of a 3D cone in shape as opposed to a 2D circle. The shape of this bleached cone changes depending on the numerical aperture of the objective used, and this needs to be accounted for as the bleached zone is complex in shape.
Some fluorescent proteins, such as GFP, have the ability to switch between a dark non-fluorescent state and a fluorescent state, with a blinking effect seen. GFP can spend time in the dark state independently of the bleaching laser, and this should be accounted for as some molecules can turn back on if they were dark while bleached. In order to account for variations in FRAP, it is important to repeat FRAP on the same spot as a control, but this can be challenging as the fluorescence is lower each time.
Cameras for FRAP
The most important thing to measure in FRAP is the fluorescence intensity. This starts high, is reduced to near zero, and then recovers to a point lower than at start. This means a sensitive camera is needed in order to best track these intensity changes with accuracy, a camera with a high signal-to-noise ratio would be ideal in order to measure these changes quantitatively.
In addition, as the events being imaged are often dynamic diffusion events across a predetermined space, a suitable camera could narrow the field of view (FOV) to the bleached region and the surrounding cell, this would also drastically improve the camera speed and allow the tracking of fast molecular events. Overall, a sCMOS camera with high speed and high sensitivity is a must for FRAP.
Summary
FRAP is a versatile biophysical technique that is widely used to study dynamics in almost all aspects of cell biology. By bleaching part of a cell, many factors can be studied, including membrane dynamics, molecular diffusion between cellular compartments, and how different cells transfer molecular signals between or within themselves. With careful experimental design and a sensitive low-light camera, FRAP is an experimental platform that can deliver quality images and data, and is an important fluorescence technique in biophysical imaging.
References
Axelrod D., Koppel D.E, Schlessinger J., Elson E. & Webb W.W (1976) Mobility measurement by analysis of fluorescence photobleaching recovery kinetics. Biophysical journal, 16(9), 1055-1069. https://doi.org/10.1016/S0006-3495(76)85755-4
Dundr M, Hoffmann-Rohrer U, Hu Q, Grummt I, Rothblum LI, Phair RD, Misteli T (2002) A kinetic framework for a mammalian RNA polymerase in vivo, Science, 22;298(5598):1623-6
Groeneweg F.L, van Royen M.E, Fenz S, Keizer V.I, Geverts B, Prins J, de Kloet E.R, Houtsmuller A.B, Schmidt T.S & Schaaf M.J (2014). Quantitation of glucocorticoid receptor DNA-binding dynamics by single-molecule microscopy and FRAP. PloS one, 9(3), e90532. https://doi.org/10.1371/journal.pone.0090532
Ishihama Y., Tadakuma H., Tani T. & Funatsu T (2008) The dynamics of pre-mRNAs and poly(A)+ RNA at speckles in living cells revealed by iFRAP studies. Experimental cell research, 314(4), 748-762. https://doi.org/10.1016/j.yexcr.2007.10.023
Ishikawa-Ankerhold H.C., Ankerhold R. & Drummen G.P (2012) Advanced fluorescence microscopy techniques-FRAP, FLIP, FLAP, FRET and FLIM. Molecules (Basel, Switzerland), 17(4), 4047-4132. https://doi.org/10.3390/molecules17044047
Tran-Ba, K.H, Higgins D.A & Ito T. (2015) Fluorescence Recovery after Photobleaching and Single-Molecule Tracking Measurements of Anisotropic Diffusion within Identical Regions of a Cylinder-Forming Diblock Copolymer Film. Analytical chemistry, 87(11), 5802-5809. https://doi.org/10.1021/acs.analchem.5b01041
Want To Learn More?
Back To Imaging Topics
Book A Camera Course
Knowledge and Learning Hub