Brillouin Microscopy
Introduction
The mechanical properties of cells and tissues play an important role in cellular function and disease development. However, standard methods for probing these mechanical properties are mostly invasive and limited to the sample's surface. This is the application suited for Brillouin microscopy, a form of imaging that takes advantage of a physical phenomenon known as Brillouin light scattering.
Brillouin microscopy is a type of optical elastography and is a non-destructive and label-free way to investigate the viscoelastic properties of biological samples in 3D.
Brillouin Light Scattering
Named after the French physicist Léon Brillouin, Brillouin light scattering (BLS) is a physical phenomenon that was first reported (in 1922) to occur when light interacts with material and undergoes scattering. All solid materials are made up of atoms and structures that are constantly vibrating but remain in fixed positions in relation to each other. Waves of vibration constantly run through solids, especially crystalline solids. These elastic vibrational waves can cause light to scatter in different ways when it interacts with a solid material.
The vibration can be modified by the following factors:
- Heat: BLS is thermally-induced and depends on the temperature of the material.
- Compression/decompression: these are acoustic waves and change depending on how much the material is compressed/decompressed. This is how sound works, as the air itself is compressed and vibrated in order to produce sound.
- Material stiffness: measured by the Young's modulus (E), BLS can report on the E of cells and tissues.
- Hydration: the water content of the material also affects the scattering, with cells and tissues often being highly hydrated
BLS is essentially the interaction of light and a solid substance, where the level of the vibration in the solid material depends on several factors, and different levels of vibration change how the light is scattered. This scattering is inelastic, meaning that the photons of light may lose or gain energy as they are scattered. This energy comes in the form of quasiparticles, such as phonons (from acoustic waves), polaritons (from charge displacement) or magnons (from oscillation spin). If scattering caused a photon to lose energy, a quasiparticle is generated (causing a Stokes shift), and if a photon gained energy it is because a quasiparticle was absorbed (causing an anti-Stokes shift), as seen in Fig.1.
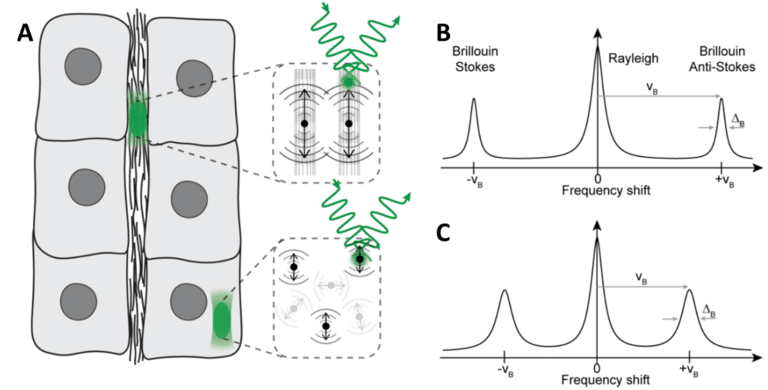
Figure 1: BLS in biological samples using a laser. A) A set of six cells and the extracellular matrix (ECM) between them. The cells and ECM
have different mechanical properties. Brillouin scattering is observed from the ECM (seen in B) and an intracellular region (seen in C). B)
Brillouin scattering from ECM, showing a gain in energy (anti-Stokes shift). C) Brillouin scattering from the cytosol, showing a smaller gain
in energy.
This shift in photon energy corresponds to a measurable Brillouin shift in frequency, meaning that BLS can measure the energy, wavelength, frequency, or speed of photons that have interacted with a solid substance. These measurements can be done with Brillouin spectroscopy and/or Brillouin microscopy.
Brillouin Microscopy
Brillouin microscopy allows for imaging of the viscoelastic and mechanical properties of 3D biological materials in a non-contact, label-free manner, including biomechanical assessment in several fields including cancer biology, tissue regeneration in zebrafish and 3D mapping of the lens corneal biomechanics. As seen in Fig.1, BLS can be used to show that solid components (ECM, Fig.1B) experience a greater shift than the cell interior (cytosol, Fig.1C) which is mostly liquid and therefore a less rigid material.
In the case of biological samples, the typical frequency shift is a few GHz. For instance, a typical Brillouin shift would be approximately 8 GHz. This is based on the sound speed in water (~1500 m/s) and visible light with a wavelength of 500 nm.
Applications Of Brillouin Microscopy
Brillouin microscopy has attracted interest in several fields including medical research as a tool for investigating the evolution of certain diseases. For instance, one of the first applications in the field of biomedicine was on the human cornea during the development of keratoconus (KC), a pathological condition that can result in cornea thinning and deformation of the lens (Yun et al. 2018). Due to the non-invasive nature of Brillouin microscopy, it is an ideal tool to understand the development of this pathology and therefore improving the diagnosis at its early stage, as seen in Fig.2.
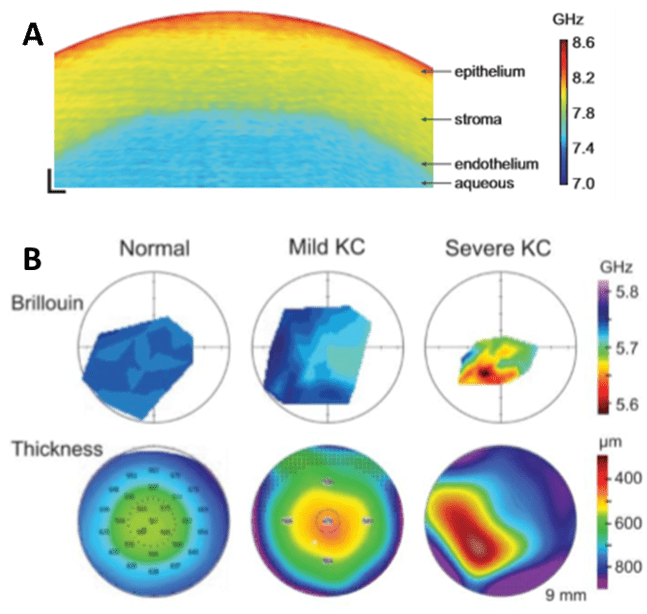
Figure 2: Studying the cornea of the eye using Brillouin microscopy. A) A cross-sectional Brillouin image of bovine
cornea, showing the decreasing stiffness with depth. Scale is 5 mm (X) and 0.5 mm (Y). Image adapted from
Scarcelli et al. (2012). B) Characterization of patients with keratoconus (KC) using Brillouin. Maps of the cornea
from healthy (normal), mild and severe KC can be compared. Colored bars correspond to Brillouin frequency
shifts in GHz. Image adapted from Yun et al. (2018).
Cancer research is another field that benefits from Brillouin microscopy. It has been reported that biomechanical properties are important in the metastatic cancer pathway, and during metastasis cancer cells are able to escape from a primary tumor and spread to other locations. In other words, for the metastatic cells to be able to migrate to other locations in the body, they have the advantage of mechanically softening. Taking advantage of Brillouin microscopy, the difference in stiffness between non-tumorigenic and metastatic breast cells can be measured, as seen in Fig.3.
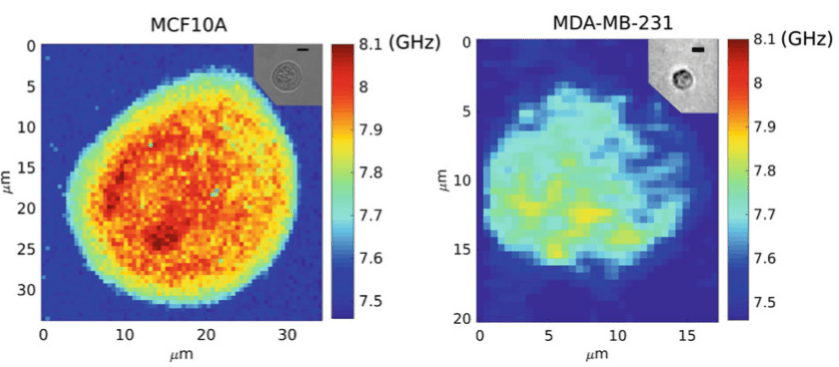
Figure 3: Brillouin microscopy comparing the stiffness of non-tumorigenic and a metastatic (tumor-forming) cells. The intracellular stiffness of two epithelial cell lines: non-tumorigenic MCF-10A (left) and metastatic MDA MB-231 (right). Insets show the related bright-field images; scale is 10 μm. The metastatic cell is appreciably softer than the non-tumorigenic cell. Image adapted from:Nikolić et al. (2018).
Brillouin microscopy is often used to measure biomechanical properties of cells and also entire embryos, as seen in Fig.4, which also compares Brillouin microscopy to traditional imaging techniques such as brightfield (Fig.4A).
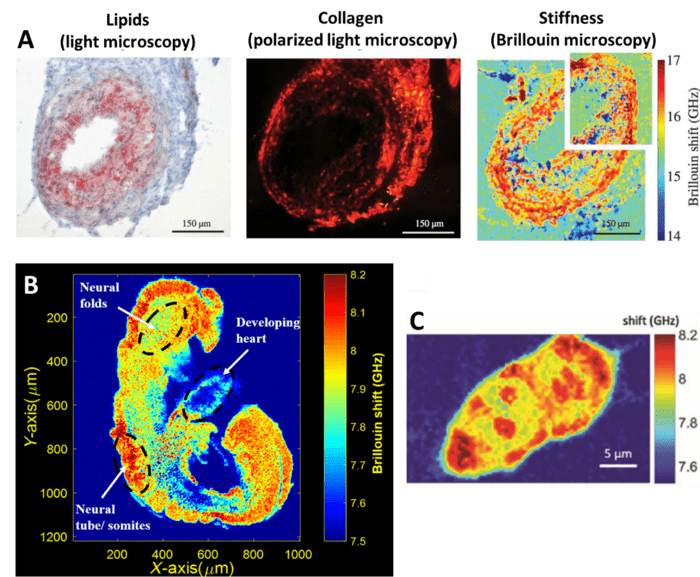
Figure 4: Brillouin microscopy of cells and embryos. A) A single cell imaged with light microscopy (highlighting lipid deposits), polarized light
microscopy (highlighting collagen), and Brillouin (highlighting stiffness). Adapted from Antoacci et al. (2015). B) A 2D elasticity map of a
plane from a whole mouse embryo, showing the stiffness of different areas. Adapted from Raghunathan et al (2017). C) Mouse embryonic
stem cell showing sub-cellular scale stiffness differences. Adapted from Prevdel et al (2019).
Brillouin can also be coupled with confocal microscopy by using a two-stage virtually imaged phased array (VIPA) spectrometer with a laser source. The illumination light can pass through a half-wave plate and a polarized beam splitter which functions as controlling the intensity of the light being sent to the microscope. The Brillouin shift can give a good approximation for the longitudinal modulus by itself. The Brillouin shift can be used as a metric of mechanical properties in the case of zebrafish embryos, as seen in Fig.5.
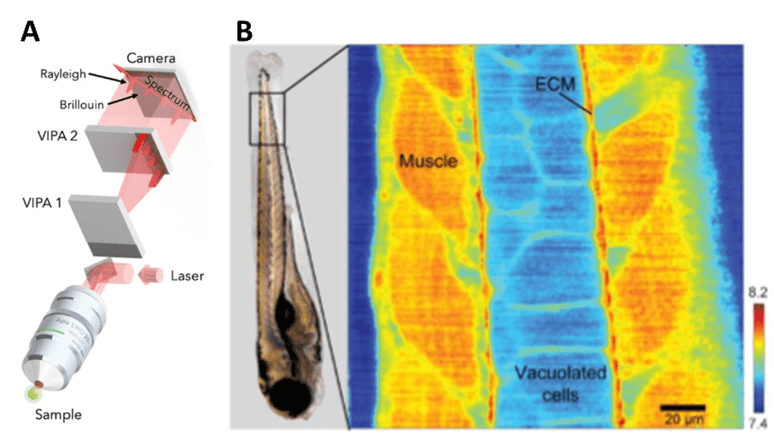
Cameras For Brillouin Microscopy
Detector cameras for Brillouin are often paired with spectrometers so that separate spectral components can be detected. A highly sensitive camera, such as a back-illuminated sCMOS with a high quantum efficiency (QE), would collect this filtered signal from the spectrometers. Live Brillouin imaging would benefit from a high-speed camera that featured a large field of view so that larger samples could also be sensitively imaged.
Summary
Brillouin spectroscopy was firstly introduced and widely used in the context of material sciences, where it became a powerful method for studying of condensed matters. Brillouin microscopy has been recently introduced in the field of mechanobiology as a tool to measure the mechanical properties in biology. Brillouin microscopy is a label-free, contact-free, and non-destructive method that can be used to study the viscoelastic properties of biological samples in 3D. Using this method, we can investigate the stiffness of cancer tissues, eye diseases, and different structures in zebrafish. Brillouin microscopy and spectroscopy can be combined with spectroscopy in order to image the stiffness of tissues. To facilitate this, we can benefit from using a high-speed CMOS camera with high quantum efficiency and high frame rates.
References
Antonacci G, Pedrigi RM, Kondiboyina A, Mehta VV, de Silva R, Paterson C, Krams R, Török P. (2015) Quantification of plaque stiffness by Brillouin microscopy in experimental thin cap fibroatheroma. J R Soc Interface. 2015 Nov 6;12(112):20150843. doi: 10.1098/rsif.2015.0843. PMID: 26559685; PMCID: PMC4685854.
Bevilacqua, C., Diz-muñoz, A., & Prevedel, R. (2019). Brillouin microscopy - measuring mechanics in biology using light, (53).
Nikolić, M., Conrad, C., Zhang, J., & Scarcelli, G. (2018). Noninvasive imaging: Brillouin confocal microscopy. Advances in Experimental Medicine and Biology, 1092, 351-364.
Prevedel, R., Diz-Muñoz, A., Ruocco, G., & Antonacci, G. (2019). Brillouin microscopy - a revolutionary tool for mechanobiology?, 1-19.
Raghunathan R., Zhang J., Wu C., Rippy J., Singh M., Larin K.V., Scarcelli G. (2017) Evaluating biomechanical properties of murine embryos using Brillouin microscopy and optical coherence tomography, J. Biomed. Opt. 22(8) 086013 https://doi.org/10.1117/1.JBO.22.8.086013
Scarcelli, G., Pineda, R., & Yun, S. H. (2012). Brillouin optical microscopy for corneal biomechanics. Investigative Ophthalmology and Visual Science, 53(1), 185-190.
Yun, S. H., & Chernyak, D. (2018). Brillouin microscopy. Current Opinion in Ophthalmology, 29(4), 299-305.
Want To Learn More?
Back To Imaging Topics
Book A Camera Course
Knowledge and Learning Hub