Ultra-High-Speed, Time-Resolved SRS Spectroscopy in Combustion
Novel(3D) Neutron Imaging Techniquefor Nondestructive Testing Made Possible by Large-Area,Intensified CCD Camera System
Introduction
In combustion, until recently only two temporal optical gating schemes were available to increase signal-to-noise ratio (SNR) for time-resolved spontaneous Raman scattering (SRS) spectroscopy. Problematic optical background noise could be rejected either byelectronic gating with an image intensifieror by using a mechanical shutter. Unfortunately, each of these traditional approaches has its shortcomings.
Image intensifiers, for example, provide excellent optical background noise rejection via <2 nsec gating capability but carry several inherent limitations, such as lesser image quality lower dynamic range. On the other hand, while a;high-speed mechanical shutter with a rotary optical chopper is able to deliver wider dynamic rangewithout diminishing the quantum efficiency (QE) of the detection system 's CCD, its30 Hz speed and ~10 µsec gating are not sufficient for rejecting noise and can result in transmission losses of up to 50%.
In 2010, Dr. Jun Kojima of the Ohio Aerospace Institute, working with Dr. David Fischer and Dr. Quang-Viet Nguyen (NASA Glenn Research Center), described an architecture* for SRS that employs a frame-transfer CCD operating in a subframe burst-gating mode to realize time-resolved combustion diagnostics.1 This patented technique enables all-electronic optical gating at microsecond shutter speeds ( <5 µsec) without compromising optical throughput or image fidelity. Dr. Kojima uses a Princeton Instruments ProEM® electron-multiplying CCD (EMCCD) camera for this method.
When utilized in conjunction with a pair of orthogonally polarized excitation lasers, the technique described above measures single-shot vibrational Raman scattering that is minimally contaminated by optical background noise. Nonetheless, its relatively long gating;(~5 µsec) still leaves room for improvement in terms of optical background rejection.
Recently, Dr. Kojima has developed another advanced technique for measuring time-resolved SRS spectroscopy in combustion (see Figure 1). An overview of this new approach, which offers even higher SNR and permits ultra-high-speed observation of combustion dynamics, is provided herein.
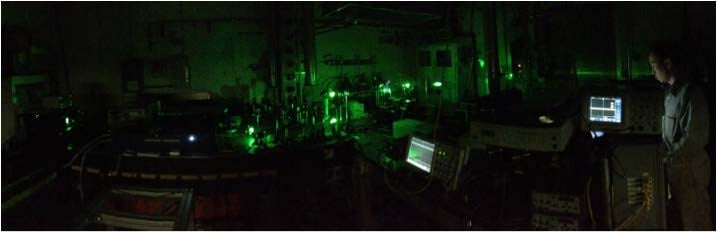
Figure 1.Dr. Jun Kojima of the Ohio Aerospace Institute testing newtechnique at NASA Glenn Research Center.
New Way To Measure Time-Resolved
Spontaneous Raman Scattering
Dr. Kojima 's new experimental apparatus is shown in Figure 2. It measures Raman scattering with much faster gating ( <2 nsec), wider dynamic range, and higher sensitivity in combustion than previously reported techniques, allowing observation of flame instability dynamics via a newly introduced Princeton Instruments intensified emICCD camera that keeps up with the latest 10 kHz lasers.
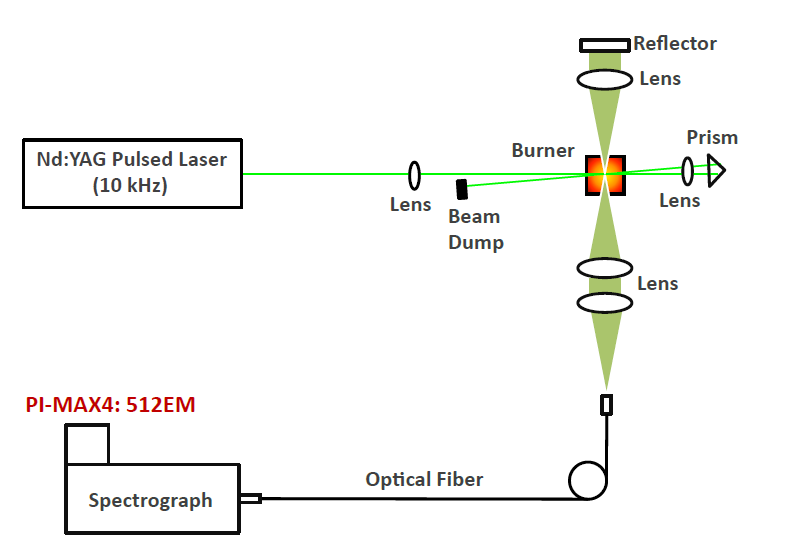
Figure 2. Experimental apparatus showing a high-speed laser Raman
diagnostic system employing an Nd:YAG pulsed laser (532 nm, 88 nsec pulse width,
10 kHz repetition rate) and a Princeton Instruments PI-MAX4:512EM camera
coupled to a lens spectrograph. Courtesy of J. Kojima (OAI/NASA).
Here, a second-harmonic Nd:YAG pulsed laser was used as an excitation source (200 W) and operated at a 10 kHz repetition rate to interrogate a flame. The scattering light was collected by fiber-coupled lens optics and transmitted to a volume-transmissive lens spectrograph equipped with a PI-MAX®4:512EM camera from Princeton Instruments.
To enhance the SNR, the image intensifier was operated at a 10 kHz rate to keep pace with the high-speed laser and gated at 90 nsec to cut out the optical flame emission background, while the EMCCD was operated at a rate of 1 kHz (i.e., 10 laser-shot accumulation) using a special feature within Princeton Instruments LightField® software that enables customization of CCD size and readout speed. This custom detection setting effectively enabled the diagnostic system to achieve the highest signal level ever achieved in the NASA facility without sacrificing the necessary kHz data rate.
Enabling Technology
New PI-MAX4 emICCD (see Figure 3) cameras from Princeton Instruments leverage the key advantages of both EMCCDs and ICCDs by fiber optically coupling an EMCCD to an image intensifier. This innovative emICCD technology lets the new cameras deliver an unrivaled combination of precision, true single-photon detection, intelligence, and speed
Figure 3. The new Princeton Instruments PI-MAX4:512EM is the first scientific
camera on the market to utilize revolutionary emICCD technology. The PI-MAX4:512EM
uses a front-illuminated EMCCD; the PI-MAX4:512EMB uses a back-illuminated EMCCD.
The PI-MAX4 emICCD camera 's back-illuminated EMCCD boasts 95% peak QE for the highest signal throughput of any ICCD camera. Furthermore, coupling the EMCCD to an image intensifier via fiberoptics delivers 6x higher light throughput between the image intensifier and the detector than lens-coupled configurations. As a result, emICCDs provide the highest signal-to-noise (SNR) of any gated imaging and spectroscopy detectors.
The exceptional linearity and dynamic range of these new emICCD cameras, achieved by intelligently programming gains between the image intensifier and the EMCCD, are critical for quantitative imaging and spectroscopy applications such as combustion. Their true single-photon detection capability, meanwhile, ensures the high sensitivity needed for light-starved applications. An oscilloscope-like user interface (see Figure 4) even remembers complete experimental setups. In addition, thanks to the ability to acquire 10,000 spectra/sec when operated in a special custom chip mode, Princeton Instruments ' emICCD cameras can capture every pulse from next-generation lasers.
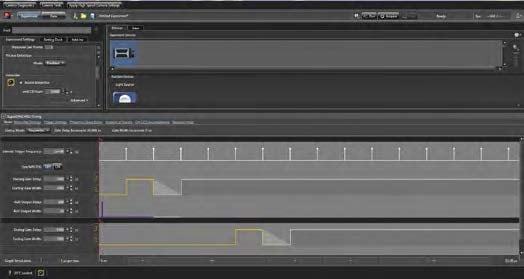
Figure 4. An oscilloscope-like user interface enhances the utility of Princeton Instruments ' emICCD cameras.
Results
High-speed laser spectroscopy measurements were performed using the diagnostic apparatus shown in Figure 2 at the Atmospheric Pressure Combustion Diagnostics (APCD) lab, NASA Glenn Research Center, in Cleveland, Ohio. Figure 5a shows a close-up of a flame. Figure 5b presents data for temporal variation of spontaneous Raman scattering of combustion species (oxygen, nitrogen, and water vapor) measured at the tip of a fuel-lean hydrogen-air flame. Pixel region (1 to 512) corresponds to the wavelength region of 486 to 680 nm.
Figure 5a. Close-up of flame.Figure 5b. Time-series Stokes Raman scattering spectra recorded at a sampling rate of 1 kHz over one second in an oscillating fuel-lean hydrogen-air flame using the high-speed Raman diagnostic apparatus with PI-MAX4:512EM. Courtesy of J. Kojima and D. Fischer (OAI/ NASA).
The signal visibility in Figure 5b is significantly higher than previously reported data of this kind. It is clearly seen from the data that the flame oscillates at a certain frequency (here, ~46 Hz) due to an interaction of the flame with ambient air entrainment.
A careful observation reveals that the pure rotational band is in inverse correlation with the O2 and N2 spectra. This is explained by the fact that the pure rotational band is a flame marker (high temperature) in contrast to the two species, which decrease their peak intensity at higher temperature. Higher concentration of water vapor (H2O), the combustion product, appears when the flame is observed. It is significant that flame dynamics were characterized in a species-resolved fashion using high-speed Raman spectroscopy.
Future Directions
The recent use of a new diagnostic apparatus to measure the dynamics of each individual molecular species, as opposed to simply acquiring bulk information (e.g., pressure), points to the possibility of performing temperature and frequency analyses of species in combustion. Ultimately, such potential applications could become diagnostic tools for fuel-air ratio dynamics at different temperatures and pressures.
Advances in scientific detector technology, such as the PI-MAX4 emICCD camera 's ability to gate out all optical background noise at <1 nsec and thus improve SNR for time-resolved spontaneous Raman scattering spectroscopy in combustion, continue to extend the horizons of research in this area.